- Assistant Professor, Chemical Engineering
- Joined College of Engineering faculty in 2021
What is your area of expertise?
My research focuses on the characterization of soft materials, which includes anything that is squishy or moves when you poke it. These materials range from those with industrial applications, such as shampoo and toothpaste, to those found in biology. You don’t want to put shampoo in your hand and have it run off like water. You want it to be thick enough to allow you to manipulate it and apply it. Our bodies often demonstrate a similar soft material behavior.
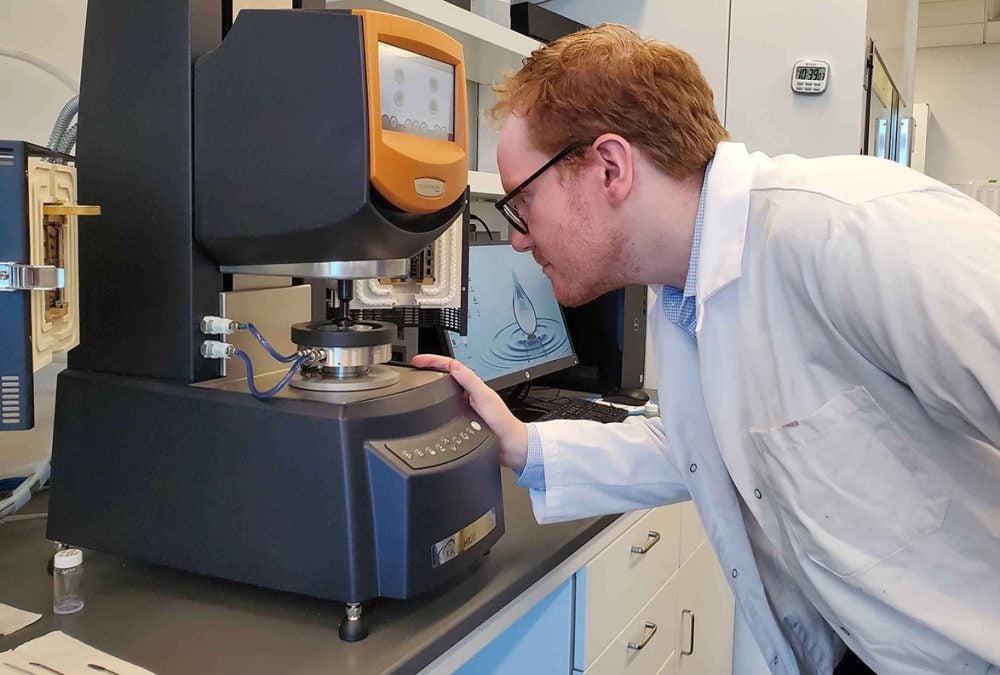
I’m focused on producing some of the biological responses that we observe in living materials into synthetic and soft materials. That includes actions such as self-assembly. Proteins in our cells can grow and divide. Can we achieve some of that behavior in synthetic materials, where we don’t have to worry about feeding it or keeping it alive or anything like that? My goal is to design materials that exhibit these types of responses.
Why are you so interested in soft materials?
I enjoy breaking down complex problems into individual components. I don’t know anything more complex than the human body, or any biological system for that matter. I want to dive into that complexity and distill it down into simple physical principles. Can we build a simple model that exhibits a certain type of behavior? We’re not trying to recreate biology. We’re trying to identify key characteristics that underlie biological behavior.
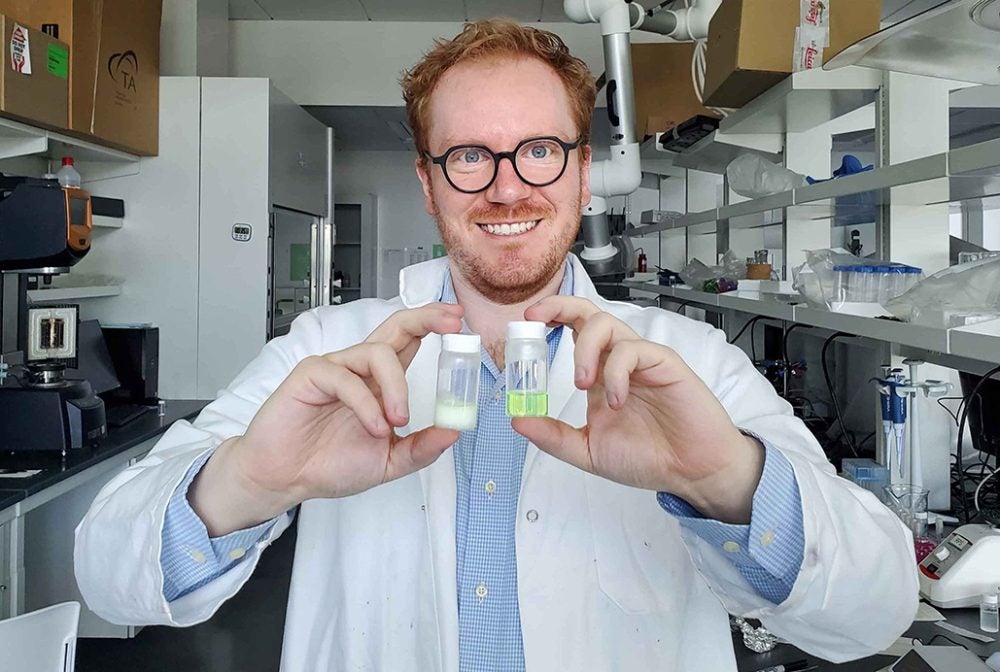
We then want to make progress on being able to manipulate soft materials as well as our body can. Scientists have worked with solid materials, such as metals and ceramics, to create amazing things. But when it comes to materials that are soft and squishy, we haven’t been able to create anything that can do what our biological system can.
What are the potential benefits of conducting research on soft materials?
Once we have systems that can behave like biology, we can use them as an intermediate step in transitioning things such as therapeutics or healthcare behaviors into clinical applications. If someone designs a new drug and wants to test whether it can transport through the body effectively, they would have to move to animal models to test the efficacy. Animal studies can be time-intensive, costly and they require a lot of work. If we can create materials that behave similarly to the body, you can put the drug in this material to see how it behaves, and then determine if it makes sense to proceed to an animal model. In theory, we can save a lot of time and money by studying the behavior in some of these soft, idealistic types of models.
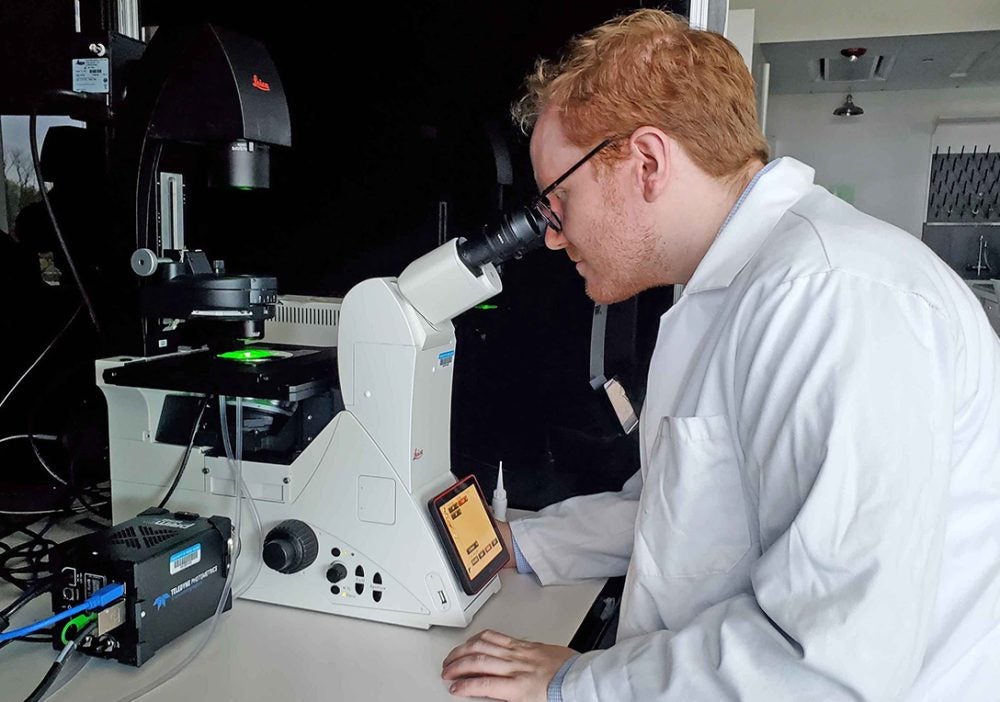
We can also gain a lot of physical insight into soft matter systems by trying to create these biological responses. Biology is incredibly good at assembling materials into a hierarchy of structures. Something on a nanoscale can build up into something larger, which can build into something even larger. For example, we have cells assembling into tissues and organs. Humans can’t really do that because our manufacturing is top-down. What we can learn from studying this assembly is how to control that hierarchical structure and how we can create new materials that address critical problems, such as energy transport and storage, water purification, cancer therapeutics, and novel sensors, all by manipulating the fundamental physics that we elucidated from biological systems.
What technology do you use for your research?
Because we deal a lot with fundamental questions, most of the work in our lab is based on characterization of methods. There is a ton of work done with synthesis and making new materials. We use those materials to understand why they have specific properties.
Our lab has two primary instruments – a rheometer that we use to test material properties and a high-speed camera attached to a microscope that allows us to measure dynamics in materials. When you’re working with materials, you often measure their structure and that can tell you about their properties. The complication with soft materials is that you have to account for dynamics. Things move because temperature causes vibration that builds up to large-scale motion.
I’m also a big scatterer. Scattering means you shoot light at an object to see how it bounces off. How the light interacts with the material tells you about the material structure. We do that with light, X-rays and neutrons. We have a light-scattering system that we use in one of the core labs, and we’re asking the National Science Foundation to fund an X-ray scattering beam line. Currently, the only way we can really do that is to go to national labs, which is inconvenient. For neutron scattering, we need a nuclear reactor, so we partner with national facilities for access to that equipment.
The materials we’re designing could potentially be 3D-printed. If we are trying to create something that looks like a tissue, we can print an artificial tissue to mimic something found in biological materials. None of the materials we create are going into the body, but they can inform us about how materials in the body behave.
Who would you like to collaborate with on projects?
Most problems need some sort of interdisciplinary work. I would like to work with Dr. Daniel Roxbury on how to transport carbon nanotubes through some of our synthetic systems because he’s using them as biological sensors. If we can understand how they move through some of our model systems, we can learn how they can transport better through biological systems.
I want to work with Dr. Geoffrey Bothun on nano particle sensors. By controlling the interactions and assembly of objects, we can use them as sensors.
Dr. Jyothi Menon is looking at using hydrogels for drug delivery. I would like to work with her to better understand how the properties of the hydrogels behave.
Because I’m a fundamental researcher, I enjoy a variety of applications. Anything that needs some of these softer, stretchier materials is something that I’m open to collaborating on.
Why did you decide to work at URI?
The facilities at URI’s College of Engineering are incredible. The collaborative nature of the chemical engineering department felt welcoming. You can work with anyone you want to. Everything is open and shared, especially in the new engineering building. The labs are interconnected. Graduate students who need training can easily find other students to help them.
URI is also in the center of a ton of academic research, not just on campus, but in the region. We have amazing institutions within driving distance that can facilitate a lot of collaborations.
How do you like working with students on research projects?
I enjoy mentoring students. We just got our first paper accepted by an academic journal. A first-year graduate student did amazing work and we were able to get it published quickly. I enjoy the enthusiasm undergraduates have for research. I encourage them to get as much exposure to research as possible
What do you like to do outside of work?
I really enjoy cooking and baking. I try to avoid making the same thing repeatedly.
There is a lot of chemistry in baking. When I bring baked goods into the classroom, I show students that they encounter some of the things we talk about in chemical engineering all the time. We think of science as a logical and defined medium, but the best science you can do involves creativity. There’s so much that we don’t know and can’t predict. Go explore and if what comes out is terrible, at least you tried it.