Elucidating nanoparticle-cell membrane interactions
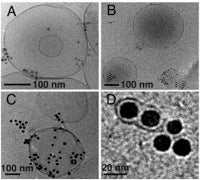
Synthetic and biomimetic lipid bilayers (~5 nm thick; as vesicle or liposomes, and supported bilayers) are being used to determine how engineered inorganic nanoparticles bind to cell membranes and alter membrane mechanics, thermodynamics, and transport. These properties are examined by cryogenic transmission electron microscopy (cryo-TEM, see image), calorimetry, and spectroscopy. The emphasis on physical nanoparticle-cell interactions represents a departure from previous approaches that have focused on biological processes, in vitro, and in vivo studies. We examine how nanoparticle composition, shape, size, and surface chemistry can lead to membrane stabilization or destabilization via lipid restructuring, membrane domain formation, and membrane curvature.
Collaborators
Christopher Kitchens, Department of Chemical and Biomolecular Engineering, Clemson University
Robert Hurt, School of Engineering, Brown University
Kai Loon Chen, Department of Geography and Environmental Engineering, Johns Hopkins University
Funding
National Science Foundation, RI-INBRE National Institutes of Health, URI Council for Research
Designing novel stimuli-responsive theranostics
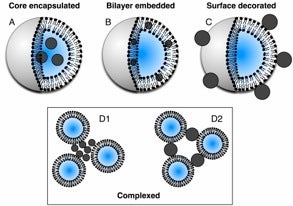
Liposomes are being used as soft scaffolds to organize and direct the assembly of hydrophobic and hydrophilic nanoparticles via physical or covalent binding strategies. Stimuli-responsive nanoparticles are used, such as superparamagnetic iron oxide or gold, that can be “activated” in by external electromagnetic fields. This provides a means of controlling LNA structure and function for therapeutic application. In addition to studying the underlying mechanisms that govern structure and function, the LNAs are designed with the goal of achieving multiple therapeutic objectives, such as cellular targeting and multi-drug delivery.
Collaborators
Arijit Bose, Department of Chemical Engineering, University of Rhode Island
Christopher Kitchens, Department of Chemical and Biological Engineering, Clemson University
Ruitang Deng, Department of Biomedical and Pharmaceutical Sciences, University of Rhode Island
David Worthen, Department of Biomedical and Pharmaceutical Sciences, University of Rhode Island
Niall Howlett, Department of Cell and Molecular Biology, University of Rhode Island
Ed Walsh, Associate Director in MRI Physics, Institute for Brain Science, Brown University
Funding
National Science Foundation, Rhode Island NASA Space Grant Consortium
Nutrient-doped nanoparticles for improving oil dispersion and biodegradation
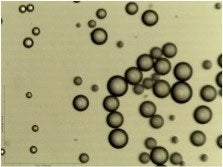
Oil spill mitigation via naturally-occurring biodegradation is dependent on nutrient availability and oil emulsion stability. Nutrient-doped nanoparticles may address both needs by creating extremely stable Pickering emulsions and providing high local nutrient concentrations. Working with the Consortium for the Molecular Engineering of Dispersant Systems (C-MEDS), mesoporous silica nanoparticles are being investigated as new dispersants capable of high nutrient loading and controlled nutrient release.
Collaborators
Arijit Bose, Department of Chemical Engineering, University of Rhode Island
Vinka Craver, Department of Civil and Environmental Engineering, University of Rhode Island
Srinivasa R. Raghavan, Department of Chemical and Biomolecular Engineering, University of Maryland
Anubhav Tripathi, School of Engineering, Brown University
Funding
Consortium for Ocean Leadership, Gulf of Mexico Research Initiative
Determine bacterial membrane response to biofuel production
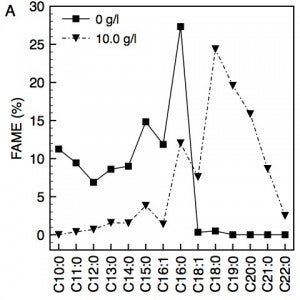
Butanol is an alternative biofuel and a platform chemical for biorefining. However, when produced by fermentation, butanol concentrations are low due to low butanol tolerance of microorganisms. Low tolerance is attributed in large part to butanol fluidization or destabilization of cellular lipid membranes. We are currently working on a n-butanol production by Clostridium pasteurianum using crude glycerol as a substrate (crude glycerol is a waste byproduct from biodiesel production). Our research examines changes in lipid composition based on ‘homeoviscous response’ to n-butanol, where lipid tail length and degree of tail saturation are altered to counteract fluidization. These results are paired with biophysical studies on model and reconstituted membranes to gain insight into the membrane biophysics associated with n-butanol tolerance.
Collaborators
Carmen Scholz, Department of Chemistry, University of Alabama in Huntsville
Lenore Martin, Department of Cell and Molecular Biology, University of Rhode Island
Funding
National Science Foundation
Creating responsive and antifouling surfaces
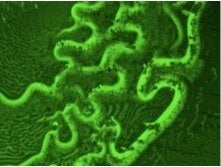
This area is pursued on two fronts. First, nanostructured polymer brush surfaces are designed using commodity polymers. The goal is to achieve antifouling properties via nanoscale topology coupled with controlled surface repulsion. Second, responsive surfaces are designed by embedding electromagnetic field-responsive nanoparticles within thermally-responsive polymer coatings. In this case, topology (e.g. coating thickness) and hydrophobicity is controlled by nanoparticle activation.
Collaborators
Jahn R. Torres, Naval Undersea Warfare Center, Newport, RI
Isabel Escobar, Department of Chemical and Environmental Engineering, University of Toledo
Funding
Naval Undersea Warfare Center/Center of Excellence in Undersea Technology