By Ellen Liberman
On a warm November morning the oaks are shedding their leaves and acorns along the winding trails of the Grills Preserve. The 544-acre tract lies south of the Pawcatuck River, which curls around the old Bradford Printing and Finishing Plant to the east. From 1790, when the first mill rights were established, to 2011, when the plant’s last owners shuttered it for good, the textile industry once thrived along this river.
Hikers can still see the vestiges of this past in the two-mile stretch of manmade waste lagoons along the riverfront, designed by the URI engineering school in the 1950s to degrade and oxidize the dyes and other organic waste before they discharged into the Pawcatuck. Today, these still-green ponds, curtained by phragmites, are home to largemouth bass, sunfish, bluegill, and pickerel. But, they aren’t safe for fishing; the mill left other remnants that aren’t visible: Per- and Polyfluoroalkyl Substances (PFAS).
PFAS refers to a class of synthetic chemicals, characterized by their common carbon chains and the presence of fluorine. PFAS’ near-unbreakable carbon-fluorine bonds renders them stable, non-reactive, and versatile. Since the 1940s, manufacturers have been exploiting PFAS’ properties to produce non-stick, waterproof, and stain-resistant products – from cosmetics to electronics to cookware to fire-fighting foam – to name a few. But, PFAS’ resistance to degradation has also made these “forever chemicals” an ubiquitous environmental and public health threat, migrating into water, air, soil — and us. PFAS chemicals are found in the blood serum of 98 percent of Americans. They are toxic at high and even extremely low levels. Studies have linked PFAS to a range of adverse outcomes, including cancer, decreased fertility and immune system function, developmental delays in children, thyroid disease and liver damage.
“We have a better understanding of the vast scope of the problem.”Professor Rainer Lohmann
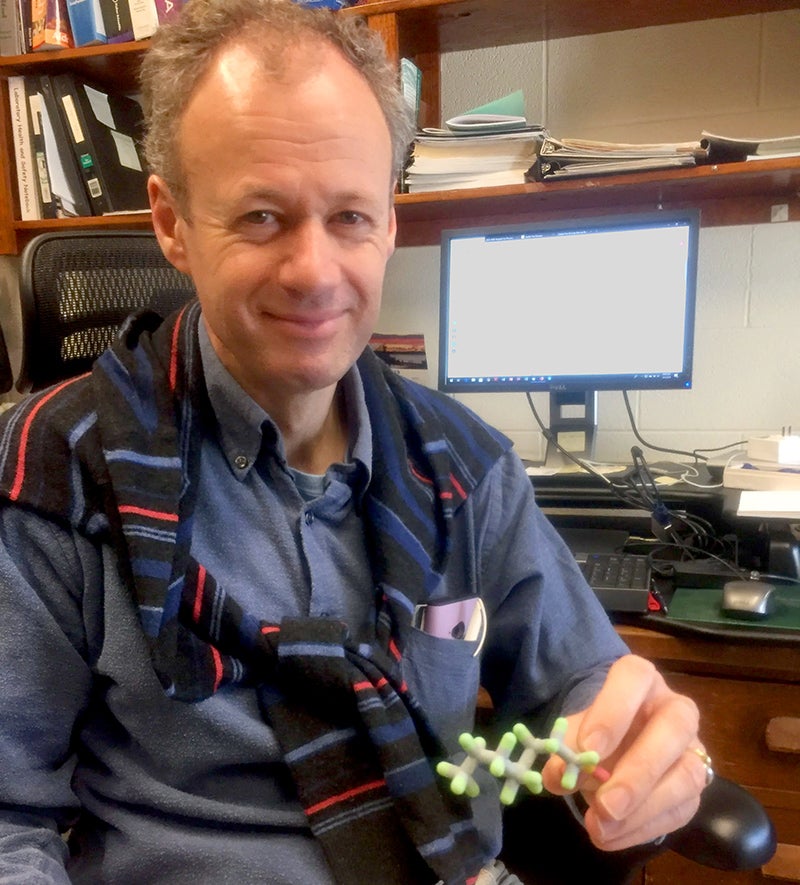
“We have a better understanding of the vast scope of the problem,” says Professor of Oceanography Rainer Lohmann, who began researching PFAS’ impact a decade ago. “What has really changed is that it has gone from being an almost niche topic to what feels like the biggest driver of contaminant research in the last few years.”
In 2021, URI returned to the Pawcatuck River to measure what those waste lagoons never filtered out. Working with the state’s Office of Land Revitalization and Sustainable Materials Management, Matt Dunn, Ph.D. ’23, deployed a type of passive water sampler he had developed in Lohmann’s lab at seven sites along the river, from Bradford to the mouth of the Atlantic Ocean.
“My focus was on the detection of PFAS in the environment. Where are PFAS in the water? Where are they coming from? Where are they going? How did they get there? How do you trace the flow of them?” he says. “One of my specific tasks was to actually develop a piece of technology that would get as much good information as we possibly could in a manner that was relatively easy and affordable because science can be really expensive.”
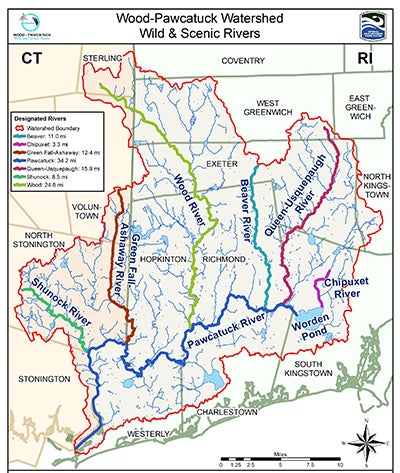
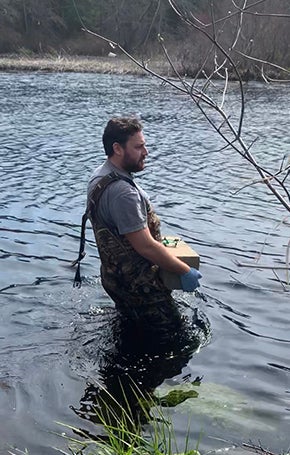
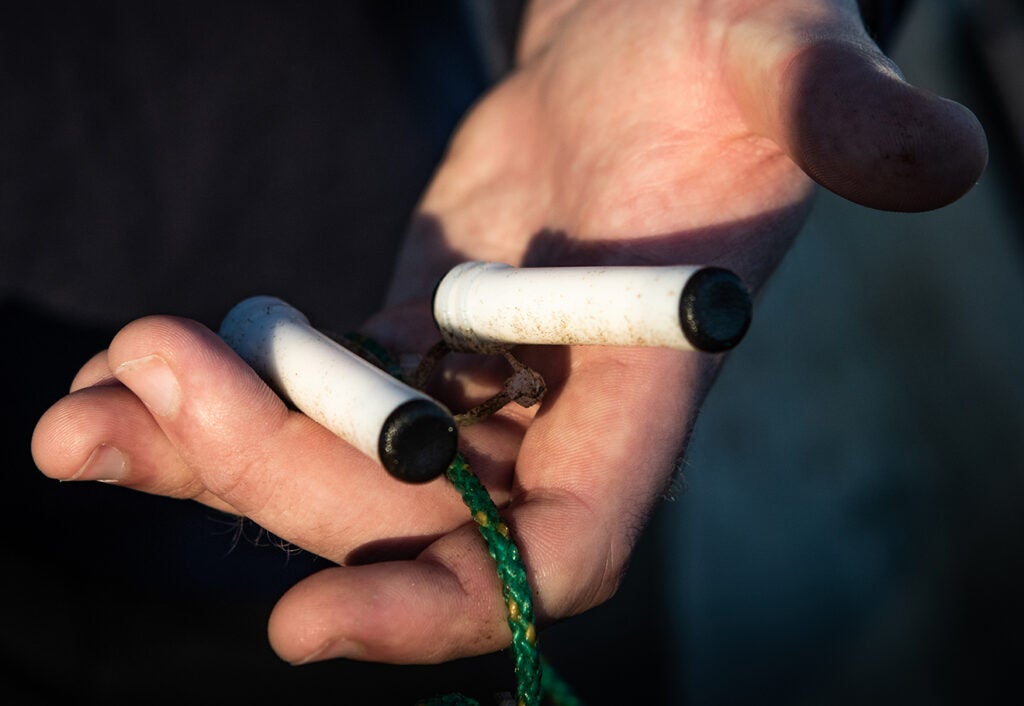
The result was a seven-centimeter-long microporous polyethylene tube housing a commercial sorbent, which could detect PFAS in ground and surface water for weeks at a time. Instead of taking discrete water samples to amass a series of PFAS snapshots in surface water, this passive sampler could quantify the flux of PFAS and distinguish different chemical fingerprints in historical effluents, as well as those still coming from textile finishing plants upstream.
Dunn’s project is among many produced by the Sources, Transport, Exposure & Effects of PFAS (STEEP) Superfund Research Program at URI. In December 2017, Lohmann and his co-director Philippe Grandjean, a URI College of Pharmacy adjunct professor and researcher, launched STEEP with a 5-year, $8.5 million grant from the National Institute of Environmental Health Sciences’ (NIEHS) Hazardous Substance Basic Research and Training Program, known as the Superfund Research Program. The program specifically funds academic research focused on “practical solutions” to hazardous substances exposures. In partnership with the Harvard T.H. Chan School of Public Health’s Department of Environmental Health and the non-profit Silent Spring Institute, STEEP aims to address how it permeates the environment, and how it affects human health
In the last seven years, STEEP has been working with researchers in the U.S. and abroad, and state and federal environmental and health agencies. Two focus areas have been monitoring the travel of PFAS used in fire-fighting foams at Joint Base Cape Cod into drinking water, and an epidemiological study in the Faroe Islands of human PFAS exposure through consumer products and dietary consumption of pilot whales. One of the program’s biggest successes has been the development of different types of passive samplers, which have been deployed in the U.S. and abroad. In 2022, the NIEHS renewed its support for STEEP with another five-year, $8.1 million award.
Improving Technology
“[Homeowners could] capture the wide scope of PFAS that are in the atmosphere and calculate concentrations very easily.”Melissa Woodward, Ph.D. Candidate
The Narragansett Bay Campus has become a nexus for talented minds who will confront PFAS in the coming decades. At GSO, several STEEP trainees—Ph.D. students and postdoctoral fellows—and faculty are working in two areas: 1) developing, refining, and adapting passive sampling technology to accurately measure PFAS’ unique properties in air, water, sediment and biota, and 2) exploring the movement of PFAS through aquatic food webs.
Ph.D. candidate and STEEP trainee Melissa Woodward has been working on a novel passive air sampler that also employs a small tube, but contains a resin sorbent, XAD. The project’s target was to create an easy-to-use device.
“People could deploy it in their own homes, offices, or somewhere else for us. And at the same time, be able to fully capture the wide scope of PFAS that are in the atmosphere and be able to calculate concentrations very easily,” says Woodward, whose work on this sampler will continue under a two-year U.S. Geological Survey grant. “That was the goal, because nothing like that really existed before.”
Snook’s sampler has been floated in the Pawcatuck River, as well as in waterways in Minnesota and Michigan for the U.S. Environmental Protection Agency.
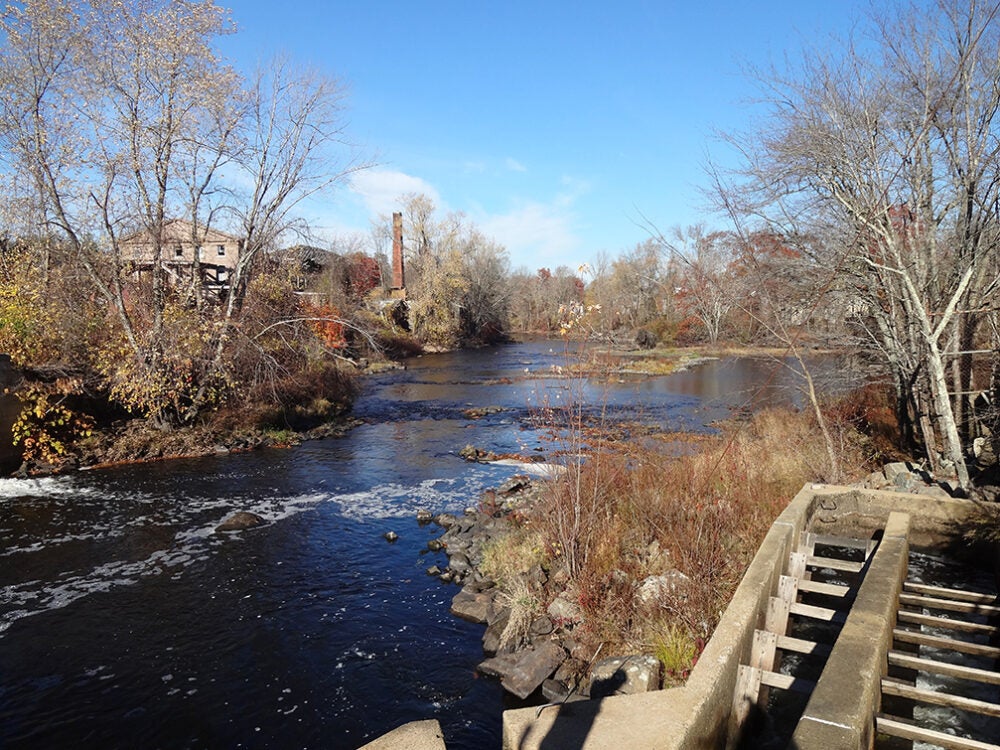
Ph.D. candidate and STEEP trainee Jarod Snook has been customizing another type of water passive sampler. Set in a small plastic frame, it employs an outer layer of agarose hydrogel, allowing PFAS to diffuse through to the sorbent layer in the middle, which captures the PFAS. Anchored by a rope to a weight or tied to a structure, like a bridge or dock, Snook’s sampler has been floated in the Pawcatuck River, as well as in waterways in Minnesota and Michigan for the U.S. E.P.A., and in Maine via a collaboration with the University of Maine.
“The rate that PFAS can diffuse through the outer layer of the gel is how we can calculate the water concentration,” he says. “This type of sampler was created in the 1990s to measure things like mercury in water, but we do have to redo the validation for new compounds like PFAS.”
These samplers have already yielded applicable results.
“We found tens to hundreds of grams of PFAS that were reaching the Atlantic Ocean from the Pawcatuck River,” says Dunn. “And 10 grams might not sound like a lot to most people, but, to put it in perspective, we consider four nanograms per liter to be a concerning level of PFAS in drinking water. That’s equivalent to about four drops of water in an Olympic-size swimming pool. For a small river, it’s a significant amount of PFAS.”
And for the Rhode Island departments of environment and health, this information will help form the foundation of future regulations. Rhode Island began to surveil the presence of PFAS in drinking water eight years ago, in response to a 2016 U.S. E.P.A. Lifetime Health Advisory setting a combined lifetime level of 70 parts per trillion for PFAS. Lohmann was among those advising state officials on which compounds should be monitored and which systems were most likely contaminated, says Nicholas Noons, a supervising engineer with R.I.’s Department of Environmental Management. The Pawcatuck River project provided “a very useful and important data set.”
“We know textile finishing and PFAS go hand-in-hand as one of the main industrial sources, and we had some spot data here or there, but with this kind of comprehensive study showed us the overall impacts to the river and the actual levels along the whole length of the waterway. It helped us issue advisories for the consumption of fish in the waste lagoons, because we can look at the surface water levels in addition to fish tissue sampling,” he added. “And, when we start looking at discharge permits, having time weighted average data on the overall PFAS loading in these rivers, will it help us determine: what’s an acceptable discharge here?”
Into the Biosphere
STEEP’s second research area is also trail blazing on how to reveal pathways and fates of PFAS in the marine food web, from the lowest to the highest trophic levels—from phytoplankton to polar bears.
Jitka Becanova, an analytical chemist and assistant research professor, who came to GSO from the Czech Republic in 2017 as a postdoctoral fellow, has been working on a passive sampler to measure PFAS in shellfish. Working with other research scientists at Brown University and in the Czech Republic, Becanova is testing nanomaterials that mimic the tissues of marine animals to create a device that can be deployed in marine environments, then analyzed to accurately measure the PFAS uptake of organisms.
“Parts of the sampler would represent different parts of the organism. After analyzing what’s in the sampler, we can understand what PFAS are in the biota, because we know that biota accumulate different PFAS differently,” she says.
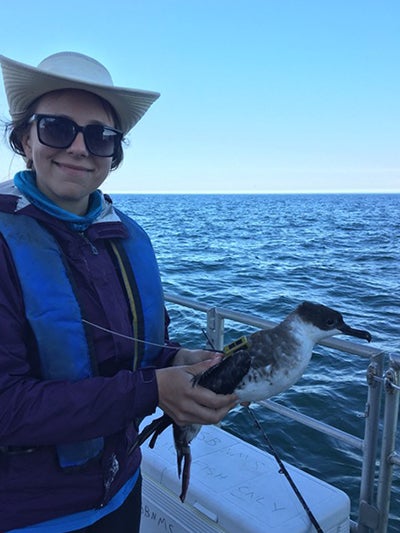
Anna Ruth Robuck, Ph.D. ’20, began to investigate PFAS in 2015 as a graduate student, studying its presence in the tissues of East Coast juvenile seabirds, including Great Shearwaters. Using tandem mass spectrometry and high-resolution mass spectrometry techniques, Robuck found PFAS levels suggesting that blood, liver, kidney and lungs are primary reservoirs. She continues this work as a principal investigator, leading a six-member research team at the EPA’s Atlantic Coastal Environmental Sciences Division.
“Ecosystems are subject to low level, but chronic exposure to a diverse array of PFAS, and the implications of that are hard to measure, because fish and wildlife are not well understood at a biological level.
We don’t have a close eye on all of the potential stressors they’re subjected to, so teasing out the impact of just one can be a challenge,” she says. “We have to use reductionist approaches to get at that question. Some of those approaches have suggested that animals with a higher PFAS exposure have different lipid profiles compared to those with less. I find that interesting, considering fat metabolism is a key factor for reproduction, for migration, and other important ecological functions.”
Ph.D. candidate and STEEP trainee Asta Habtemichael is conducting lab-based experiments and examining environmental samples, from the Gulf of Maine, among other locations, to determine the uptake mechanisms of PFAS in the lower trophic levels. Habtemichael, working with other GSO phytoplankton ecologists, is maintaining monocultures of two phytoplankton and two zooplankton species to understand when equilibrium of partitioning between the water and the biological organism occurs, and the different PFAS concentration dependencies. The goal is to derive the bioaccumulation factor.
“We are trying to understand where that story of PFAS in marine food webs begins.”Asta Habtemichael, Ph.D. candidate and 2025 John A. Knauss Fellow
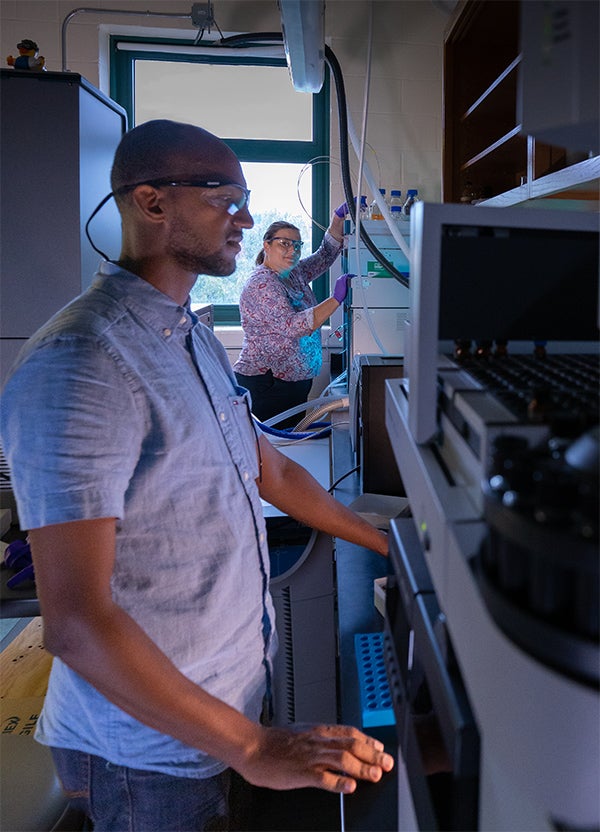
“We know from the literature how PFAS binds to the tissues and organs of the higher trophic organisms, and that it’s there in higher concentrations. We are trying to understand where that story of PFAS in marine food webs begins. So, that is going one level down from the environmental plankton data that we have, and looking at the phytoplankton and zooplankton separately.”
In April, the surveillance and epidemiological data resulted in the first E.P.A. regulation setting drinking water standards at about four parts per trillion for two most studied compounds, PFOA and PFOS. This represents policy and scientific advances, but there is still a long way to go. Setting maximum contaminated levels at trace amounts means that accurate and sensitive passive samplers will be critical in determining if remediations are successful.
“We don’t treat PFAS as a group, like a class of chemicals, but as individual chemicals,” Becanova says. “So we don’t know the future direction of PFAS, because we are still using them. When some PFAS are banned, industry just develops different molecules.”
For PFAS researchers, the field is exciting and daunting.
“In just the four years that I’ve been working on PFAS, the amount of progress that has happened is incredible. But there’s so much to learn all the time,” says Snook. “And, when there’s so many people working on it, finding your place as a young researcher can be intimidating. But you also get to meet all these people and so it provides really good opportunities.”
Woodward calls it “one of those ‘tip-of-the-iceberg problems’ where it probably goes a lot deeper than we realize. And the more you learn about PFAS, the more you realize there is so much more you don’t know.”