The Tiny Tech That’s Changing Everything
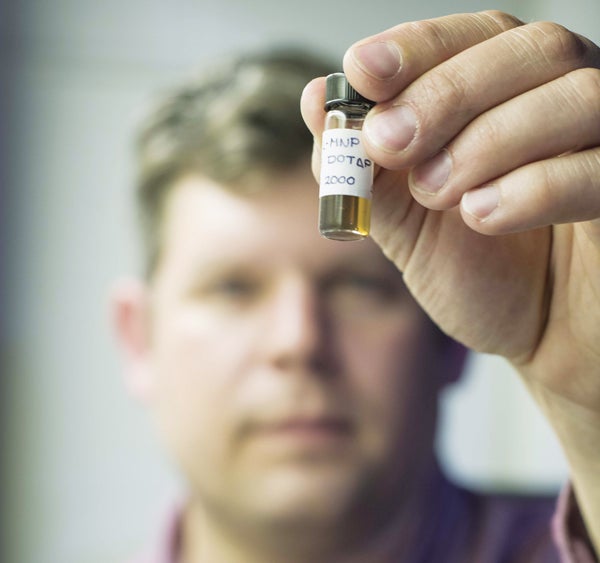
Brain cancer, oil pollution, stinky socks—the wildly exploding field of nanotechnology is taking aim at all of them, with thousands of consumer goods and medical applications already in use. Inside one URI lab, a researcher works at the frontier of our understanding of the physical world.
By Todd McLeish
Don’t tell Geoff Bothun not to sweat the small stuff, because he knows better. The popular expression suggests that we shouldn’t get stressed about the little things in life, but in Bothun’s world, focusing on the little things may be the key to improving our health, protecting the environment, keeping us safe and living longer.
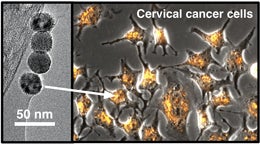
The URI professor of chemical engineering studies how to use nanotechnology to manipulate cells and molecules, enabling engineers to design materials, products and processes for biomedical and environmental applications like never before.
Imagine tiny particles—100 times thinner than a human hair and too small to see with a classroom microscope—that can make tennis balls last longer, keep your clothing cooler, heal wounds faster, clean diesel engine exhaust, and increase a computer’s speed and memory. In the medical field, nanoparticles can be injected into the body, pass through the circulatory system, hide from the immune system, and deliver a cargo of medicine to targeted cells.
“It’s the next industrial revolution,” says Bothun, who grew up in northern California and joined the URI faculty in 2006. “The strength of a material—a tennis racket or golf club, for instance—comes from the way the molecules and atoms within the material are interacting with each other. If you can control how those atoms form the material, you can design strength or additional functionalities into a larger-scale product.”
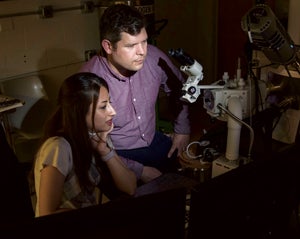
More than 1,600 consumer products use nanotechnologies in their design or manufacture. Bothun first set his sights on using nanotechnologies to improve magnetic resonance imaging (MRI), a medical diagnostics tool that uses magnetic fields and radio waves to create images of anatomy and physiological processes. He believes magnetic nanoparticles could improve the imagery by increasing the contrast and resolution between healthy and diseased cells, helping doctors detect diseases at earlier stages, and offering a general platform for improved cancer treatment.
But then a neighbor was diagnosed with glioblastoma, an aggressive brain cancer that is difficult to treat and offers little hope of a cure. Soon after his neighbor died from the disease, the sister of Bothun’s close friend was also diagnosed with glioblastoma. So he turned his research attention to improving the early detection of the devastating illness.
“Patient prognosis for glioblastoma is quite poor, with a life expectancy of only about 12 months after diagnosis, so the key to treatment is early diagnosis,” Bothun said. “We’re aiming to design something that could target glioblastoma cells and tumors in the brain, something sensitive that would allow for early detection by MRI, and carry a therapeutic cargo to the tumor right when you’re doing the detection.”
Bothun said that glioblastomas quickly develop a resistance to commonly used chemotherapy drugs, rendering them ineffective. It’s also difficult to deliver targeted treatments into the brain.
From Sunscreen to Supplements
Nanoparticles are so easy to incorporate into a wide range of materials that more than 1,600 products currently contain them, many suspended in fluids like car lubricants and skin lotion. Others are incorporated into textiles and electronics.
Tiny particles of silver are used in more than 400 different products, making it the most commonly used nanotech for consumer products. Since silver is known for its anti-microbial properties, it is used in the manufacture of athletic clothing, including socks and T-shirts, to slow the development of odor-causing bacteria. It’s also used in household disinfectants.
Nanoparticles of titanium dioxide are used in many sunscreens, while calcium and magnesium nanoparticles are often combined in dietary supplements. Other nano-substances in consumer goods: gold, carbon, copper, iron, ceramic and silicon.
Are Nanoparticles Dangerous?
Researchers are working to understand the potential pitfalls of substances like nanosilver. Washing machines coated with it, and socks and toothbrushes infused with it, can flush small amounts daily down the drain. Once in the environment, it can travel great distances. One fear: it could alter the bacteria that live in soil, disrupting natural nutrient cycles. Another: its overuse in consumer goods could allow bacteria to become resistant, crippling the effectiveness of the silver-coated instruments that surgeons rely on to keep infection rates down in operating rooms.
Another controversy: Whether the particles commonly used in sunscreen can cross the epidermal barrier, or break down in the presence of UV rays or pool chlorine to create harmful substances.
It’s an emerging field, but no strong adverse effects have so far been found. “Just because you make a material small, doesn’t mean it’s going to be dangerous,” Bothun says. But, he adds, “Their small size—sometimes on the same size scale as biological molecules—means they can interact strongly with living organisms. And determining what makes nanoparticles toxic is not trivial, because the mechanisms involved are highly complex.”
“There’s a cell layer separating the circulatory system from the brain called the blood-brain barrier, and whenever you try to deliver a therapeutic to the brain, that barrier acts as a line of defense to protect it,” he explained. “A huge challenge in the field of neuroscience and drug delivery is being able to cross that barrier.”
But researchers have found that nanoparticles with a lipid coating can make it through. So Bothun is engineering a lipid-coated nanoparticle that could be guided to diseased cells and tumors in the brain. How does that work? It comes down to how greedy cancer cells are.
“Cancer cells have a lot of growth receptors,” Bothun explains. “So if you take a nanoparticle and decorate the surface with a molecule that binds with a growth receptor, you can preferentially target the cancer cells and accumulate the drug or the imaging agent right there.”
Once near a malignancy, the particles can get in by exploiting a related weakness. “Tumors have to take up lots of nutrients so they can grow quicker than healthy tissue, so they’re a bit chaotic and have lots of leaky vasculature in them,” Bothun says. “This allows nanoparticles to migrate through the porous blood vessels and into the tumor.”
Once there, Bothun plans, they will deliver a lethal payload. Not only could the nanoparticles make the cells and tumors darker on imaging to help doctors diagnose disease sooner, but by also incorporating agents for gene therapy into the nanoparticle, Bothun plans to fiddle with their DNA, making them more sensitive to chemotherapies. Some forms of chemotherapy could be delivered at the same time, hitting the glioblastoma with a one-two punch.
Bothun knows that research of this kind takes many years of painstaking work, but he’s up for the challenge. The early stages have been successful, and in collaboration with URI Associate Professor Niall Howlett and Brown University Assistant Professor Edward Walsh, along with three URI graduate students and five undergrads, he is preparing to begin testing it on mice. “We’ve already shown that we can deliver the nanoparticles to cervical and lung cancer cells,” he says. Brain cells will come next.
But that’s not all Bothun has on his plate. The field of nanotechnology contains untold opportunities for exploration, so he is working on several other projects as well.
He recently completed a three-year study with Professor Arijit Bose to create novel approaches to cleaning up oil spills in the marine environment. Bothun’s part of the project examined how nanoparticles could be used to stop spilled oil from forming slicks on the water’s surface, where it can threaten wildlife and wash up on beaches. He found that nanoparticles of silicon dioxide used in conjunction with conventional oil spill dispersants improve the effectiveness of the dispersants.
“Dispersants work by breaking up the oil into smaller droplets that remain in the water column where they can be consumed by oil-eating bacteria,” Bothun said. “We found that those oil droplets will stick to aggregates of silicon nanoparticles, making the oil heavy enough to sink to the bottom. Having it sink into the sediment is far better than having an oil slick at the surface.”
He is continuing this line of research with funds from a new three-year grant.
Bothun also directs the Rhode Island Consortium for Nanoscience and Nanotechnology, which brings together nanotechnology researchers from URI, Brown University and elsewhere for research collaborations. The consortium has laboratory equipment and space in Crawford Hall and Morrill Hall, where local and national companies often can be found conducting analyses of their products.
In addition, Bothun has a great interest in workforce development for the nanotechnology industry, which is expected to grow to $3 trillion by 2020. “It represents the next technological revolution, so within a decade there are going to be lots of jobs in this area,” he said. “And that means we need graduates trained in how to use the instruments to characterize products containing nanotechnology.”
So where will the nanotechnology field go next?
“I expect that within 10 years, we’ll have nano-medicines that are safe, specific, personalized and smart,” Bothun said. “We’ll soon get to the point where we can design systems tailor-made for specific diseases and specific individuals.”
In the meantime, he is looking forward to a large state-of-the-art nanotechnology and imaging laboratory in the new College of Engineering building, which should break ground next year: “I’m really excited about the possibilities.” •